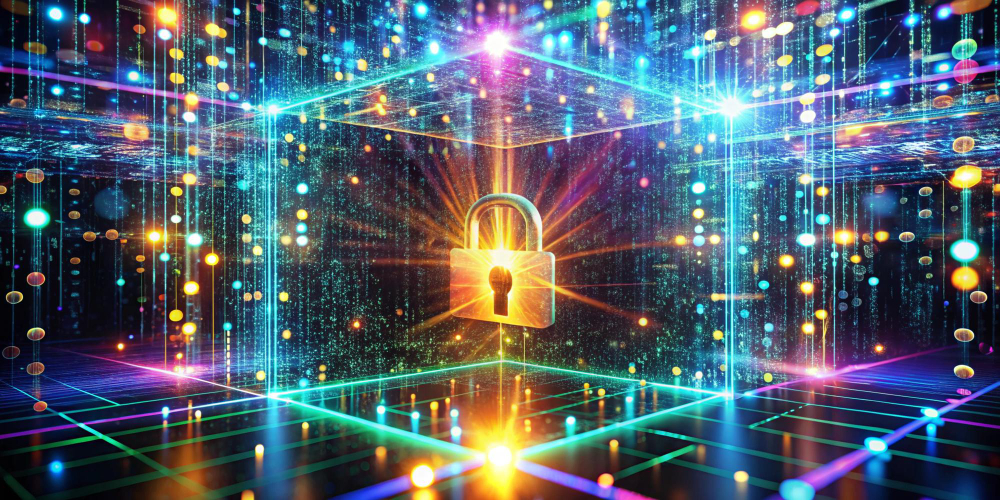
Cyber Security
Quantum Cryptography Explained: Data Security Revolution
Andrea Abbondanza ,
18 Oct, 2024
Quantum cryptography is transforming data protection by using the laws of quantum mechanics to create highly secure encryption.
It relies on quantum states, such as photon polarization, to safely exchange encryption keys between parties. This method’s unmatched security makes it a good option for safeguarding sensitive communications.
Interested in learning more? Let’s explore quantum cryptography to understand how it’s shaping the future of secure data transmission.
What is Quantum Cryptography?
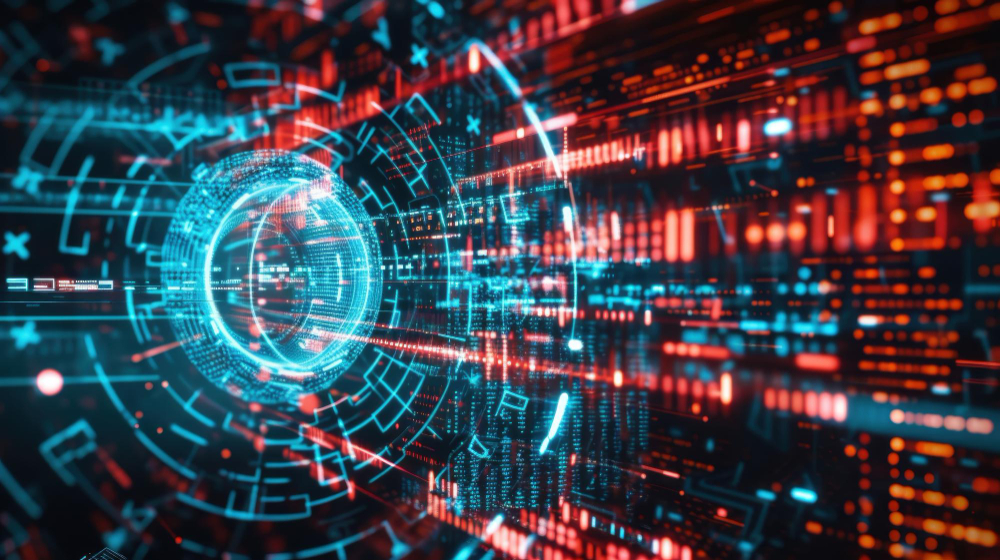
We all know cryptography secures data by making it unreadable to unauthorized users. Quantum cryptography, or quantum encryption, elevates this by applying the laws of quantum physics to create an unbreakable secret key for encryption.
This new, safer method doesn’t rely on cryptographic algorithms—just like the traditional one—but ensures that any eavesdropper is instantly detected through the principles of quantum mechanical properties.
Why is Quantum Cryptography Important?
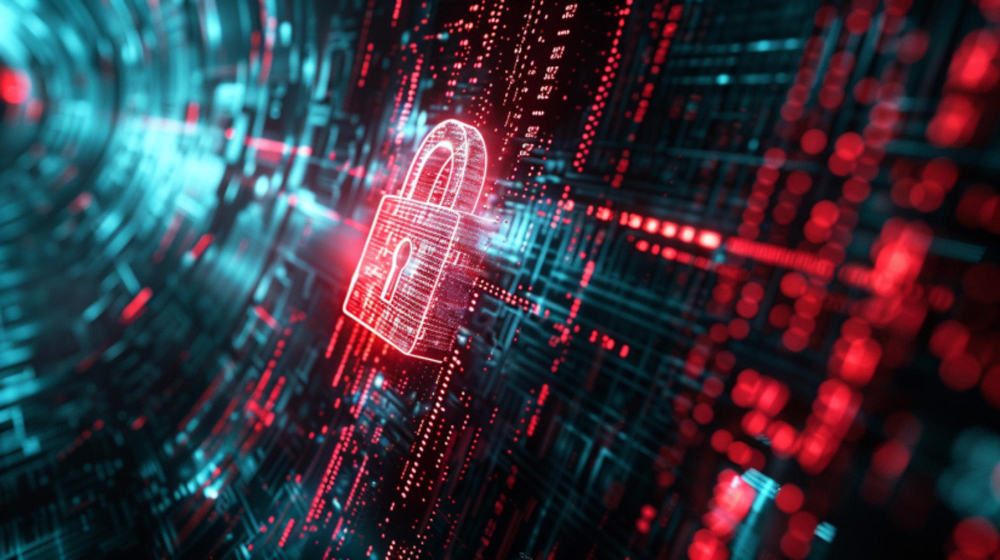
Traditional cryptography has been key for securing data, but quantum computing now poses a significant threat to these systems.
Fortunately, quantum cryptography offers a more secure solution using principles of quantum physics, such as entanglement and Heisenberg’s Uncertainty Principle.
If anyone tries to eavesdrop or intercept the data, both the sender and receiver can immediately detect it because the measurement process in quantum systems alters the state of qubits.
As a result, quantum key distribution (QKD) allows encryption keys to be transmitted in a way that cannot be intercepted without being noticed.
The whole security process makes quantum cryptography important.
How Does Quantum Cryptography Work?
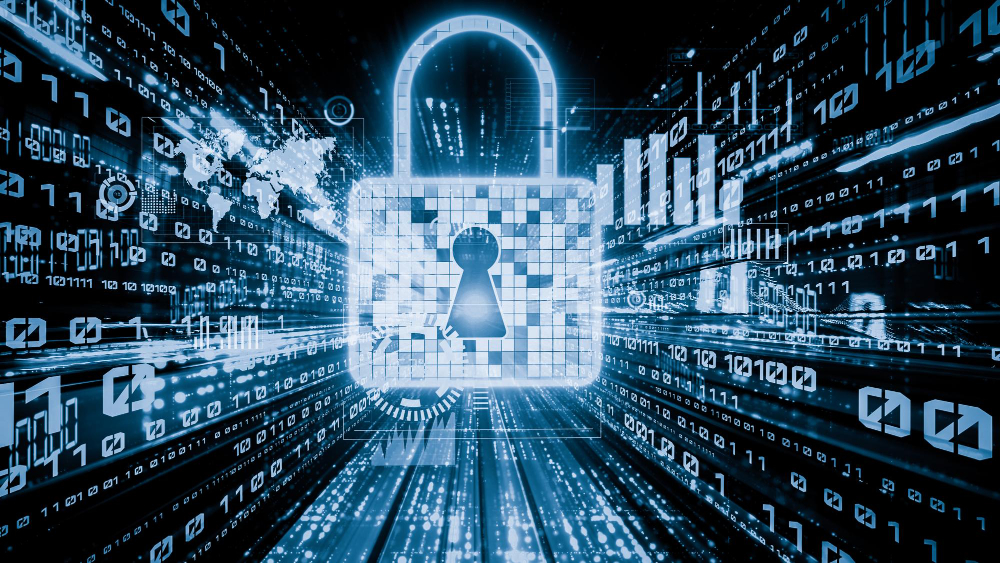
Quantum cryptography works by using photons—particles of light—to securely transmit data.
Each photon represents binary values, either 0 or 1, and travels through optic cables to carry this information.
When two parties, like Alice and Bob, exchange a secret key, the quantum cryptography protocol uses these photons in different quantum states to encrypt and decrypt the data.
If eavesdroppers try to intercept the key, the laws of quantum physics cause the photons to change, which alerts Alice and Bob to the breach.
This ensures a secure key exchange that is highly resistant to tampering or interception.
Types of Quantum Cryptography
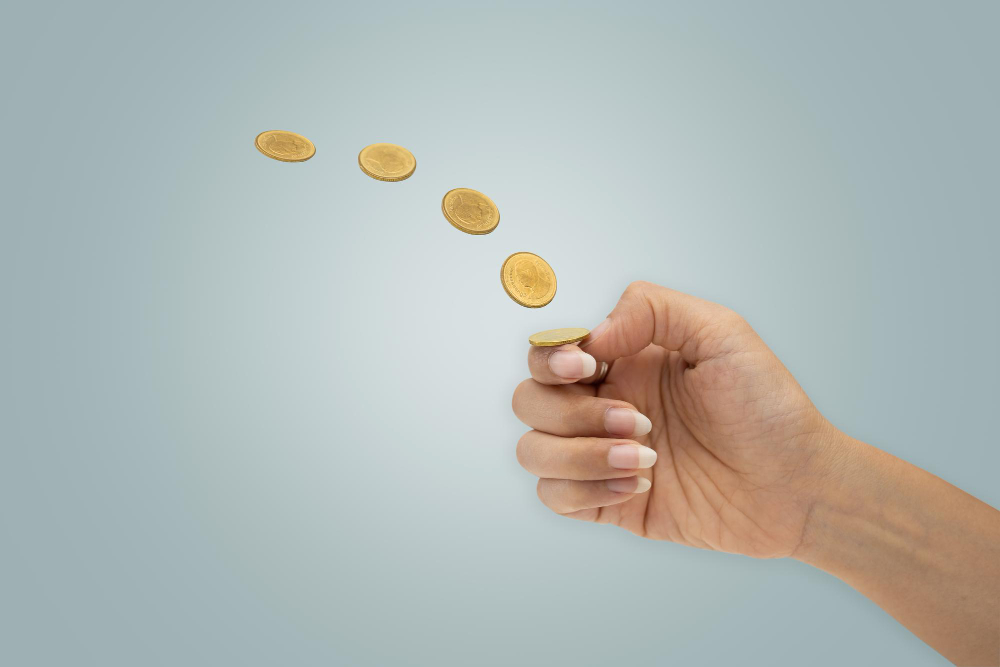
There are three major types of quantum cryptography, including:
Quantum Key Distribution (QKD)
QKD is a secure method that uses quantum mechanics to generate a secret key between two parties. It can detect eavesdropping because measuring quantum states disturbs them, revealing any interference.
Key protocols include BB84, which uses photon polarization, and E91, which uses quantum entanglement.
QKD system distributes encryption keys, which are often used with algorithms like AES encryption.
It has been tested over long distances and even via satellites, with growing commercial use for high-security needs in government and finance.
Quantum Coin-flipping
Quantum coin flipping is a cryptographic protocol that uses quantum mechanics to help two distrustful parties agree on a random outcome.
Unlike classical methods, it prevents perfect cheating because any interference with quantum states is detectable.
The protocol’s security is measured by its bias—the lower the bias, the harder it is to cheat.
There are two types: strong coin flipping, in which neither party knows the other’s preferred outcome, and weak coin flipping, in which both do.
Although quantum coin flipping offers better security than classical methods, practical implementation is challenging.
Additional Types of Quantum Cryptography
Many researchers are still studying quantum cryptography to this day. Other types include:
- Quantum Secure Direct Communication (QSDC)
- Quantum Digital Signatures (QDS)
- Quantum Byzantine Agreement
- Quantum Bit Commitment
- Quantum Secret Sharing (QSS)
- Quantum Oblivious Transfer (QOT)
- Position-based Quantum Cryptography
- Device-independent Quantum Cryptography
- Kak Protocol
- Y-00 Protocol
Differences between Traditional Cryptography and Quantum Cryptography
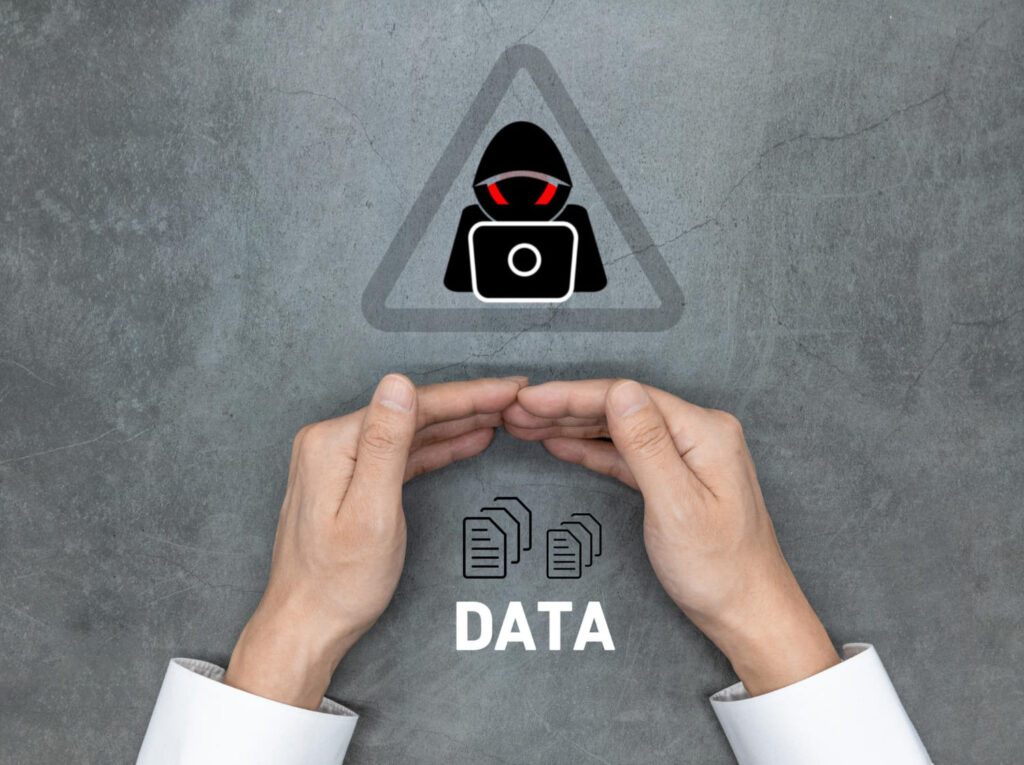
Here are the key differences between traditional and quantum cryptography based on various aspects:
- Security Basis:
- Traditional: Relies on mathematical complexity (e.g., factoring large numbers, discrete logarithms) for security.
- Quantum: It is based on the principles of quantum mechanics, offering security through the laws of physics.
- Vulnerability to Quantum Attacks:
- Traditional: Vulnerable to attacks from quantum computers (e.g., Shor’s algorithm can break RSA and ECC).
- Quantum: Resistant to quantum computer attacks, as it leverages quantum properties for secure key exchange (e.g., QKD).
- Eavesdropping Detection:
- Traditional: Cannot inherently detect eavesdropping during communication.
- Quantum: It can detect eavesdropping due to quantum measurement disturbance (e.g., Heisenberg’s uncertainty principle).
- Key Distribution:
- Traditional: Uses classical key exchange methods (e.g., RSA, Diffie-Hellman), which rely on computational hardness.
- Quantum: Uses Quantum Key Distribution (QKD), which allows two separate parties to generate and share a secure key with guaranteed security.
- Dependence on Computational Power:
- Traditional: Security decreases as computational power increases, especially with the advent of quantum computers.
- Quantum: Security is independent of computational power since it is based on quantum mechanics rather than algorithmic complexity.
- Authentication:
- Traditional: Authentication methods rely on cryptographic hash functions or digital signatures, which may become insecure with quantum attacks.
- Quantum: Typically relies on classical authentication for the communication channel, but the security of the key exchange is quantum-safe.
- Practical Implementation:
- Traditional: Widely used, mature, and easier to implement with current infrastructure.
- Quantum: Still in experimental or early commercial stages, with challenges in scalability and practical deployment (e.g., distance limitations, specialized hardware).
- Error Correction and Noise:
- Traditional: Works well over noisy classical channels with established error-correcting codes.
- Quantum: Sensitive to noise, requiring additional mechanisms like privacy amplification and error correction tailored to quantum systems.
- Cost and Infrastructure:
- Traditional: Relatively low cost, can be implemented in software and on standard hardware.
- Quantum: Requires specialized hardware (e.g., photon detectors, quantum channels), making it more costly and infrastructure-intensive.
- Scalability:
- Traditional: Scalable for widespread use across the internet and global communications.
- Quantum: Currently limited by technology, distance, and availability of quantum repeaters or satellite-based quantum communication.
These points highlight the core differences between the two cryptographic approaches across several dimensions.
Frequently Asked Questions
Is quantum cryptography possible?
Yes, quantum cryptography is feasible and has been successfully implemented, particularly through applications like Quantum Key Distribution (QKD).
What is an example of quantum encryption?
An example is the BB84 protocol, used in Quantum Key Distribution (QKD) to securely share encryption keys via quantum states.
What technology is used in quantum cryptography?
It relies on technologies such as single photon detectors, quantum communication channels (like optical fibers or free space), and quantum random number generators.
Conclusion
Quantum cryptography represents a major leap in secure communication, using quantum mechanics to detect eavesdropping and ensure unbreakable encryption.
As cybersecurity evolves, how can organizations stay ahead of these challenges? While quantum technology solutions may not yet be widely adopted, Fluxgate’s expert cybersecurity services are here to help you implement strong security strategies.
Contact Fluxgate today to strengthen your defenses and protect your data from current and future cyber threats.
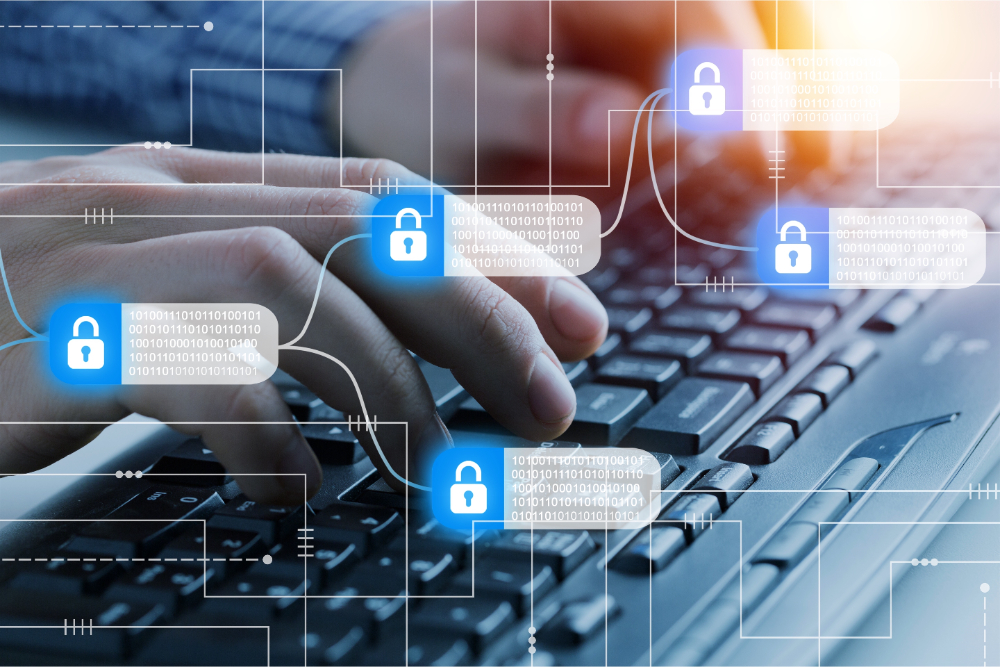